Biomechanical Study of 3 Osteoconductive Materials Applied in Pedicle Augmentation and Revision for Osteoporotic Vertebrae: Allograft Bone Particles, Calcium Phosphate Cement, Demineralized Bone Matrix
Article information
Abstract
Objective
This study assessed biomechanical properties of pedicle screws enhanced or revised with 3 materials. We aimed to compare the efficacy of these materials in pedicle augmentation and revision.
Methods
One hundred twenty human cadaveric vertebrae were utilized for in vitro testing. Vertebrae bone density was evaluated. Allograft bone particles (ABP), calcium phosphate cement (CPC), and demineralized bone matrix (DBM) were used to augment or revise pedicle screw. Post the implantation of pedicle screws, parameters such as insertional torque, pullout strength, cycles to failure and failure load were measured using specialized instruments.
Results
ABP, CPC, and DBM significantly enhanced biomechanical properties of the screws. CPC augmentation showed superior properties compared to ABP or DBM. ABP-augmented screws had higher cycles to failure and failure loads than DBM-augmented screws, with no difference in pullout strength. CPC-revised screws exhibited similar strength to the original screws, while ABP-revised screws showed comparable cycles to failure and failure loads but lower pullout strength. DBM-revised screws did not match the original screws’ strength.
Conclusion
ABP, CPC, and DBM effectively improve pedicle screw stability for pedicle augmentation. CPC demonstrated the highest efficacy, followed by ABP, while DBM was less effective. For pedicle revision, CPC is recommended as the primary choice, with ABP as an alternative. However, using DBM for pedicle revision is not recommended.
INTRODUCTION
With the increasing incidence of osteoporosis, spinal surgeons have observed a corresponding rise in the prevalence of screw loosening among patients [1,2]. Screw loosening is defined radiographically as the presence of a ≥ 1-mm clear minimum thickness zone around the pedicle screw, as visualized on computed tomography (CT) imaging, often exhibiting the “double halo sign” [3]. Screw loosening is a significant factor contributing to the formation of radiological and clinical pseudoarthrosis. To enhance the stability of screws within osteoporotic vertebrae, researchers have explored a range of methods, including pedicle screws augmentation (pedicle screws augmentation refers to the technique of implanting reinforcement material within a trajectory to enhance the biomechanical performance of a screw), improved screw shape design such as expandable screws, improved screw surface material design such as screws with uneven surfaces, and improved screw pathway direction design such as cortical bone trajectory screws [4-9]. The most commonly used method to improve screw stability is polymethylmethacrylate (PMMA) augmentation of pedicle screw. However, this material has many disadvantages, such as its toxicity [10], exothermic curing process [11,12], and the inert nature of PMMA, which prevents absorption within the body. As a result, the exploration of alternatives to PMMA has emerged as a primary research focus in the field of pedicle screw augmentation.
In a previous study [13], we found that using allograft bone particles (ABP) for pedicle screw augmentation significantly improved the biomechanical performance of the screws. Over the years, calcium phosphate cement (CPC) has been used for pedicle screw augmentation. CPC, on the one hand, offers substantial holding force for the screws [14], while on the other hand, it exhibits a high potential for bone remodeling and osseointegration due to its osteoconductivity [15,16]. Demineralized bone matrix (DBM) is produced by removing cellular and mineral components from human corticocancellous cadaver bone, leaving the extracellular matrix molecules, including bone morphogenic proteins, which are osteoinductive [17]. It has been widely investigated as a biomaterial to promote new bone formation and is utilized clinically for bone repair and regeneration. However, no study has hitherto successfully used DBM in pedicle screw augmentation.
This study represents a fundamental biomechanical experiment involving testing screws reinforced with augmentation materials on human cadaveric vertebrae. We carried out an evaluation of 3 such materials, ABP, CPC, and DBM, and their impact on biomechanical performance during pedicle screw augmentation and revision. Additionally, we conducted comparative analyses of the augmentation efficacy of these materials. The findings from this research could provide a theoretical basis for the application of these materials in clinical cases.
MATERIALS AND METHODS
1. Specimens
This study utilized osteoporotic human lower thoracic and lumbar vertebrae obtained from our university’s department of human anatomy. Ethical approval was obtained prior to the commencement of the study. One hundred twenty vertebrae from T10 to L5 were extracted from 18 adult cadavers, consisting of 8 males and 10 females. Each vertebra underwent meticulous separation from adjacent vertebrae and careful dissection to remove all soft tissues. Radiographs were taken to ensure the absence of any bony anomalies that could potentially impact the mechanical properties of the spine, such as fractures, tumors, or severe degeneration.
2. Determination of Bone Mineral Density
The bone mineral density (BMD) of vertebrae was evaluated using dual-energy x-ray absorptiometry. As a reference, osteoporotic human cadaveric vertebrae were defined as having a BMD less than 0.8 g/cm2 or a T score of 2.5 or below [18].
3. Pedicle Screw and Augmentation Materials
According to the 3-dimensional CT measurement results of the vertebrae, we selected 5.5-mm× 40-mm pedicle screw for the lower thoracic vertebrae and 6.5-mm× 45-mm pedicle screw for the lumbar vertebrae. We prepared and tapped the pedicle screw trajectory using the Weinstein’s technique method. The depth of tapping matches the planned length of pedicle screw implantation. The “original pedicle” is the initial trajectory created using the aforementioned method. After the pedicle screw is inserted, it can undergo immediate biomechanical tests, such as pullout and cyclic fatigue tests. The “augmentation pedicle” is a trajectory prepared using the same method, but with augmentation material placed into the trajectory before inserting the pedicle screw. After the augmentation material has cured, biomechanical testing is carried out. The “revision pedicle” refers to a situation in which, after biomechanical testing of the original pedicle, the screw becomes loose (in the pullout test, the screw is pulled out by 5 mm, or in the cyclic fatigue tests, the displacement of the screw tip reaches ± 2.5 mm). In such cases, the loose screw is removed, augmentation material is inserted into the loosened pedicle, and then the same size screw is implanted along the original trajectory. Subsequently, biomechanical testing is conducted. To deliver the augmentation materials, a delivery tube commonly used in kyphoplasty was utilized. The CPC (Fig. 1A) used in this experiment consisted of a powder component composed of calcium phosphate salt and a liquid curing component composed of soluble phosphate aqueous solution. These 2 components were carefully mixed in specific proportions to create a paste-like consistency. The resulting paste was then transferred to the delivery tube using an injector, enabling its application for pedicle screw augmentation and revision. We prepared and mixed the CPC, implanted it into the trajectories, and waited for 3 minutes to allow preliminary cement curing before inserting the pedicle screws. After screw implantation, we waited for at least 20 minutes to ensure full cement curing before conducting biomechanical testing. In addition, ABP with a size of 1 mm (Fig. 1B) were selected for pedicle screw augmentation and revision purposes in the study. The average BMD of ABP was 0.45 g/mL. The DBM (Fig. 1C) used in this experiment was derived from donated bone decellularized, defatted, and decalcified to retain its components. In the CPC and DBM groups, we implanted 1.5 mL of the respective material into the pedicles of each lumbar vertebra and 1 mL into thoracic vertebrae. In the ABP groups, we implanted 0.5 g of ABP into the pedicles of each lumbar vertebra and 0.3 g into thoracic vertebrae.
4. Biomechanical Experiment
A torque meter was employed to measure the torque generated during screw insertion, which was performed using a screwdriver. The maximum torque value was recorded after the completion of the insertion procedure.
Before using the biomechanical testing device, a specialized fixation device was used to fix and adjust the direction of the vertebrae [13]. Axial pullout testing (Fig. 2A) was conducted at a constant rate of 5 mm/min until the screw had been displaced 5 mm. The maximum load recorded during testing was defined as the failure peak load or pullout strength. Fatigue testing (Fig. 2B) was conducted using a sinusoidal, cyclic force with a frequency of 1 Hz. During the initial cycle, the applied load varied from -150 N to 150 N, the minimum force required to run the material-testing machine in a stable state. Compression force was incrementally increased by 25 N every 500 cycles to expedite the failure process. Testing was terminated once the cranial-caudal displacement of the load cell had reached 5 mm [13,19]. Furthermore, the number of cycles and the corresponding maximum linear force (failure load) until the end of the test was recorded.
5. Experimental Groups
One hundred twenty vertebrae were randomly divided into 12 groups (Table 1). The pullout test was performed on groups A1, B1, C1, D1, E1, and F1 (n = 12 vertebrae each). The cyclic fatigue test was performed on groups A2, B2, C2, D2, E2, and F2 (n = 8 vertebrae each).
1) Original, augmentation, and revision
Groups A, B, and C: As the original pedicle, one pedicle from each vertebra was chosen randomly. The screw was placed into the virgin trajectory in the original pedicle, and biomechanical tests (torque, axial pullout test, or cyclic fatigue test) were performed immediately. When the biomechanical testing was completed, the screw in the original pedicle was removed. The failed trajectory (revision pedicle) and contralateral pedicle (augmentation pedicle) were then filled with augmentation materials. The same amount of augmentation materials was employed in both the augmentation and revision pedicles. In each experimental group, specific augmentation materials were utilized as follows: group A employed ABP, group B utilized CPC, and group C employed DBM. Following the augmentation or revision of the pedicles, the screws were installed, and subsequent biomechanical tests were conducted to evaluate their performance.
2) ABP vs. CPC vs. DBM
Groups D, E, and F: The primary objective of these groups was to evaluate and compare the efficacy and impact of the different augmentation materials used in this study. Prior to the insertion of screws, pedicle augmentation was performed using one specific augmentation material on one side, while a different augmentation material was employed on the contralateral side. In group D, the comparison focused on ABP versus DBM. Group E compared ABP with CPC, while group F examined the contrast between DBM and CPC. After screw insertion, biomechanical testing was conducted to assess the performance and stability of the screws in each group.
6. Statistical Analysis
The data were analyzed using IBM SPSS Statistics ver. 26.0 (IBM Co., Armonk, NY, USA). Descriptive statistics encompassing frequency and mean ± standard deviation were computed. Paired t-tests were employed to assess paired variables within the same vertebra. Curve estimation regression analysis was used to analyze the relationship between revision rate (revision rate= failure loads of revision pedicle/failure loads of original pedicle) and BMD. Statistical significance was established at p < 0.05.
RESULTS
In the present study, we found that all vertebral specimens had BMD lower than 0.80 g/cm2. The grouping of all vertebrae and the mean BMD for each group are shown in Table 1.
1. ABP: Origin, Revision, and Augmentation
The detailed results of group A1 testing are shown in Table 2. Statistical comparisons (Fig. 3A) revealed that the maximum pullout force of the revision pedicle was only 80.26% of that achieved with the original pedicle (t = 3.257, p = 0.008). The maximum pullout force of the augmentation pedicle was 144.94% of that achieved with the original pedicle (t = -4.741, p = 0.001). Additionally, the maximum pullout force of the augmentation pedicle was significantly higher than that of the revision pedicle (t = 5.367, p < 0.001). The insertional torque of the augmentation pedicle was also significantly higher than that of the original pedicle (t = -5.741, p < 0.001) and the revision pedicle (t = -3.034, p = 0.011). However, there was no significant difference in torque values between the original and revision pedicles (t = 0.000, p = 1.000). The detailed results of group A2 testing are shown in Table 3. Statistical comparisons (Fig. 4A, D) revealed that the screw failure loads of the revision pedicle reached 97.93% of those achieved with the original pedicle (t = 0.911, p = 0.393). The screw failure loads of the augmentation pedicle were 123.68% of those achieved with the original pedicle (t = -6.359, p<0.001). At the same time, the screw failure loads of the augmentation pedicle were significantly higher than the revision pedicle (t = 8.802, p < 0.001). The insertional torque of the augmentation pedicle was higher than that of the original pedicle (t = -4.680, p = 0.002). However, there were no significant differences in the torque values among the other groups.
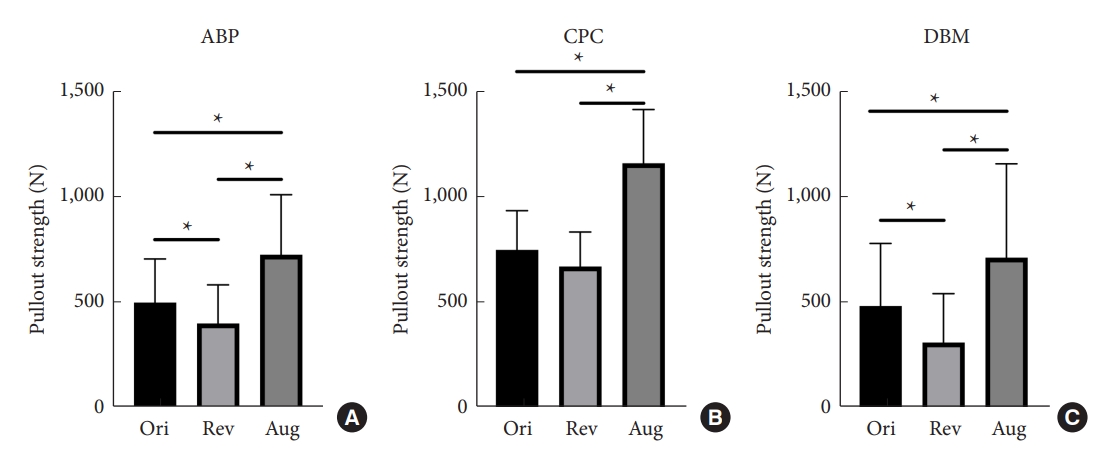
Graphs show pullout strength in groups A1-ABP (A), B1-CPC (B), and C1-DBM (C). Statistically significant differences between the 2 treatments are marked with an asterisk (*). ABP, allograft bone particles; CPC, calcium phosphate cement; DBM, demineralized bone matrix; Ori, original; Rev, revision; Aug, augmentation.
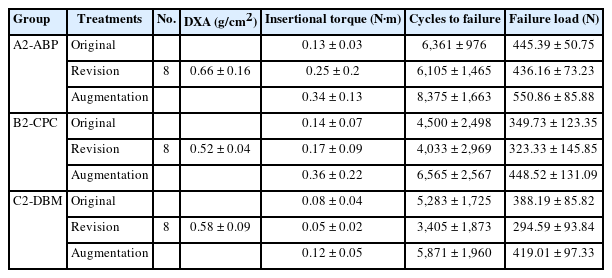
Measured insertional torques, cycles to failure and failure loads in cyclic fatigue test in groups A2, B2, C2

Graphs show cycles to failure and failure load in groups A2-ABP (A, D), B2-CPC (B, E), and C2-DBM (C, F). Statistically significant differences between the 2 treatments are marked with an asterisk (*). ABP, allograft bone particles; CPC, calcium phosphate cement; DBM, demineralized bone matrix; Ori, original; Rev, revision; Aug, augmentation.
2. CPC: Origin, Revision, and Augmentation
The detailed results of group B1 testing are shown in Table 2. Statistical analysis (Fig. 3B) revealed that the maximum pullout force of the revision pedicle could reach 89.66% of that achieved with the original pedicle (t=1.952, p=0.77). In contrast, the maximum pullout force of the augmentation pedicle was 154.14% of that achieved with the original pedicle (t = -4.744, p = 0.001). Furthermore, the maximum pullout force of the augmentation pedicle was significantly higher than that of the revision pedicle (t = -7.149, p < 0.001). The insertional torque of the augmentation pedicle was significantly higher than that of the original pedicle (t = -3.002, p = 0.012) and the revision pedicle (t = -3.397, p = 0.006). However, there was no significant difference between the insertional torques of the revision pedicle and the original pedicle (t = 0.206, p = 0.840). The detailed results of group B2 testing are shown in Table 3. Statistical analysis (Fig. 4B, E) revealed that the failure loads of the screws in the revision pedicle could reach 92.45% of those achieved with the original pedicle (t = 2.214, p = 0.062). The failure loads of the screws in the augmentation pedicle could reach 128.25% of those achieved with the original pedicle (t = -9.182, p < 0.001). At the same time, the failure loads of the screws in the augmentation pedicle were higher than in the revision pedicle (t = 7.215, p < 0.001). The insertional torque of the augmentation pedicle was significantly higher than the original pedicle (t = -3.340, p = 0.012) and the revision pedicle (t = 3.381, p = 0.012), while there was no significant difference between the insertional torques of the revision pedicle and the original pedicle (t = -2.033, p = 0.082).
3. DBM: Origin, Revision, and Augmentation
The detailed results of group C1 testing are shown in Table 2. Statistical analysis (Fig. 3C) revealed that the maximum pullout force of the revision pedicle could only reach 64.38% of that of the original pedicle (t = 3.862, p = 0.003). The maximum pullout force of the augmentation pedicle could reach 146.91% of that of the original pedicle (t = -4.386, p = 0.001). At the same time, the maximum pullout force of the augmentation pedicle was significantly higher than that of the revision pedicle (t = -5.535, p < 0.001). The insertional torques of the augmentation pedicle (t = -3.150, p = 0.009) and the original pedicle (t = 2.770, p = 0.018) were significantly higher than that of the revision pedicle. However, there was no significant difference in insertional torques between the augmentation and original pedicles (t = -1.808, p = 0.098). The detailed results of group C2 testing are shown in Table 3. Statistical analysis (Fig. 4C, F) revealed that the failure loads of the screws in the revision pedicle could only reach 75.89% of those achieved with the original pedicle (t=6.323, p<0.001). The failure loads of the screws in the augmentation pedicle reached 107.94% of those achieved with the original pedicle (t = -6.041, p = 0.001). At the same time, the failure loads of the screws in the augmentation pedicle were significantly higher than those with the revision pedicle (t = 8.370, p<0.001). Statistical analysis comparing the insertional torques of each group showed that the augmentation pedicle had the highest insertional torque, followed by the original pedicle, and then the revision pedicle and the difference among the groups was statistically significant (p < 0.05).
4. ABP vs. DBM
The detailed results of group D1 testing are shown in Table 4. Statistical analysis (Fig. 5A) revealed no significant difference in the maximum pullout force between the screws in the ABP pedicle and the DBM pedicle (t = 1.977, p = 0.074). The 2 groups had no significant difference in insertional torques (t = -0.479, p = 0.642). The detailed results of group D2 testing are shown in Table 5. Statistical analysis (Fig. 6A, D) revealed that the number of cycles (t = 6.312, p = 0.016), the failure loads of the screws (t = 7.450, p < 0.001) and the insertional torques (t = 3.149, p = 0.016) of the screws in the ABP-augmented pedicle were significantly higher than those in the DBM-augmented pedicle.

Graphs show pullout strength in groups D1-ABP vs. DBM (A), E1-ABP vs. CPC (B), and F1-CPC vs. DBM (C). Statistically significant differences between the 2 treatments are marked with an asterisk (*). ABP, allograft bone particles; CPC, calcium phosphate cement; DBM, demineralized bone matrix.

Measured insertional torques, cycles to failure, and failure loads in cyclic fatigue test in groups D2, E2, F2
5. ABP vs. CPC
The detailed results of group E1 testing are shown in Table 4. Significant differences (Fig. 5B) were found between the maximum pullout force of the screws in the CPC pedicle compared to the ABP pedicle (t = -3.505, p = 0.005). The insertional torque of the CPC pedicle was also higher than that of the ABP pedicle (t = -6.622, p < 0.001). The detailed results of group E2 testing are shown in Table 5. Significant differences (Fig. 6B, E) were found by comparing the values of the number of cycles (t=-5.534, p=0.001) and the failure loads (t=-5.509, p=0.001) of the screws in the CPC pedicle compared to the ABP pedicle. However, there was no significant difference in the values of the insertional torques between the 2 groups (t = 0.442, p = 0.672).
6. DBM vs. CPC
The detailed results of group F1 testing are shown in Table 4. Significant differences (Fig. 5C) were found by comparing the maximum pullout force (t = 3.200, p = 0.008) and insertional torques (t = 3.595, p = 0.004) values of the screws in the CPC pedicle compared to the DBM pedicle. The detailed results of group F2 testing are shown in Table 5. Besides, significant differences (Fig. 6C, F) were found by comparing the values of the number of cycles (t=-11.033, p<0.001), failure loads (t=-12.146, p<0.001), and insertional torque (t = -5.625, p = 0.001) of the screws in the CPC pedicle compared to the DBM pedicle.
7. Curve Estimation Regression Analysis Between Revision Rate and BMD
As shown in Fig. 7, in groups A2 and B2, the revision rate of both ABP and CPC were significantly correlated with BMD. The curve formulas, R2 and p-values for the fitted revision rates with respect to bone density values were as follows: groups A2 (ABP): y= -0.16/x+1.23, R2= 0.667, p = 0.013; groups B2 (CPC): y= 50.39x3-37.25x2+3.84, R2= 0.832, p = 0.012.
DISCUSSION
It has been established that screws loosening due to osteoporosis poses significant challenges for spinal surgeons during clinical practice [20,21]. Despite the drawbacks associated with PMMA, surgeons sometimes resort to using PMMA-strengthened screws for spinal internal fixation surgery [22,23]. However, to overcome the limitations of PMMA, researchers have explored various materials for pedicle screw augmentation. In recent years, several materials, such as hydroxyapatite (HA), CPC, and ABP, have been investigated for pedicle screw augmentation. There is an increasing consensus that these materials have the potential to significantly enhance screw stability [5,14,24]. Some of these materials do not release heat during curing, some are nontoxic and difficult to leak, and some have biocompatibility and osteogenicity. They provide new options for replacing the clinical use of PMMA. In a previous study [13], we documented that using ABP for pedicle screw augmentation could significantly improve the axial pullout force of screws and the failure load in cyclic fatigue experiments achieved through the study and refinement of bone grafting techniques, building upon earlier research findings [24,25]. In the present study, 2 other augmentation materials, CPC and DBM, were introduced in addition to ABP. CPC is a reinforcing material extensively studied in the context of pedicle screw augmentation [26]. Previous research has demonstrated that CPC could significantly enhance screw stability and possesses bone-forming properties [16]. DBM is a biological material known for its bone-forming properties and compatibility with bone cements [27]. DBM has been used in various applications related to bone regeneration and grafting. However, in the specific context of this experiment, it represents the first use of DBM for pedicle screw augmentation and revision.
Several important observations were made in the experiments involving different materials for pedicle screw augmentation and revision. In the ABP group, A1 and A2, the pullout force and fatigue load cycle of the augmented pedicle screws using ABP were significantly higher than those of the original screws. These results indicate that ABP could significantly improve the biomechanical properties of pedicle screws in osteoporotic vertebral bodies. However, when ABP was used for pedicle screw revision after loosening, the pullout strength of the revised screws could not reach the level of the original screws, although the fatigue load of the revised screw was comparable to the original screws. Therefore, further experiments are needed to confirm whether ABP is indicated for pedicle screw revision. The pedicle screws augmented with CPC showed significantly increased pullout force and fatigue load cycle compared to the original screws. Besides, the revised screws with CPC exhibited pullout strength and fatigue load levels similar to those of the original screws. These results suggest that CPC is an ideal material for pedicle screw augmentation and revision due to its good biomechanical performance. The pedicle screws augmented with DBM also showed increased pullout force and fatigue load compared to the original screws. However, when DBM was used for pedicle screw revision after loosening, both the pullout strength and fatigue load of the revised screws were unable to reach the level of the original screws, indicating that DBM should only be considered for pedicle screw augmentation and not for revision.
It is widely acknowledged that the fixation strength of pedicle screws is closely related to the strength of the screw-bone interface [28]. The 3 augmentation materials used in this experiment could significantly improve the strength of the screw-bone interface. Overall, we found that screws augmented with the 3 types of materials exhibited good biomechanical performance. In this respect, the pullout force of the pedicle screws in the 3 augmented screw groups could reach 144.94% (ABP), 154.14% (CPC), and 146.91% (DBM), respectively, compared to the original unreinforced screws. In other studies, various pedicle screw augmentation schemes have been investigated, revealing notable improvements in maximum pullout force. Expandable pedicle screws have been shown to increase the maximum pullout force by approximately 130%–150%. cortical bone trajectory screws have demonstrated an approximate increase of 130% in the maximum pullout force. Carbonated HA bone cement-reinforced pedicle screws have exhibited a significant increase of approximately 168% in the maximum pullout force. Moreover, PMMA bone cement-reinforced pedicle screws have exhibited the highest improvement, with an approximate increase of 202% in the maximum pullout force [29-32]. However, PMMA is essential to note some of its well-known drawbacks, including its toxicity [10], exothermic curing process [11,12], and the inert nature of PMMA, which prevents absorption within the body. In addition, PMMA has a short curing time, which means that if PMMA is used for pedicle screw augmentation, there is a limited window of time for the procedure, requiring a higher level of skill from the surgeon. Although these 3 augmentation materials in the study exhibit limited ability to yield the pullout strength achieved by PMMA bone cement augmentation technology, they still bring certain advantages compared to other pedicle screw augmentation techniques, given their bone-absorbing, tissue-compatible, and boneconductive properties, with the potential to further improve the mechanical strength of bone tissue during remodeling [16]. Animal experiments have found that bone transformation occurs after CPC implantation in vivo [26]. In addition, transverse vertebral fractures occurred during the process of augmented screws pullout (Fig. 8) in all 3 material augmentation groups, which indicated that the pullout strength of the augmented screws reached a satisfactory level. No leakage of reinforcing material into the spinal canal was observed during the experiment. At the same time, the hardening process of CPC was predominantly nonexothermic and did not cause damage to surrounding tissues [33]. Therefore, using these 3 materials for pedicle screw augmentation is a relatively safe technique for improving the stability of pedicle screws. Moreover, compared to PMMA bone cement, these 3 augmentation materials offer an additional advantage by posing less complexity in secondary revision surgeries [34].
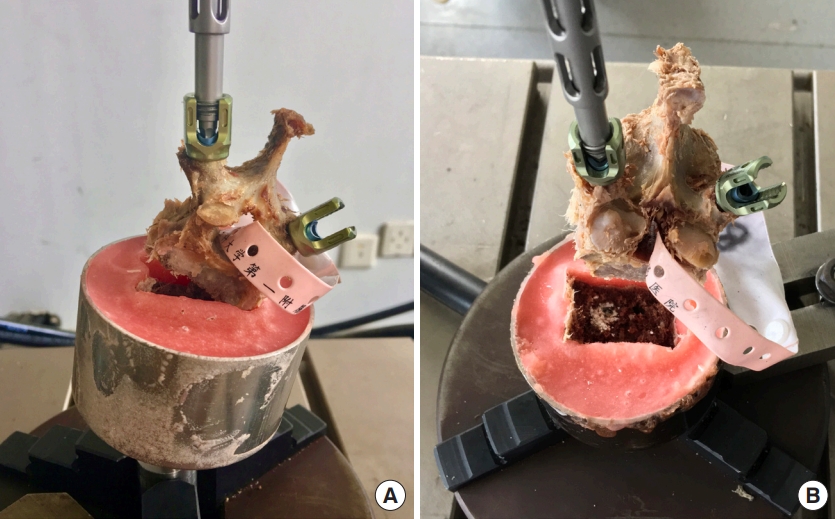
During the process of pullout test on the augmented screw, a transverse vertebral fracture (A, B) occurred.
When encountering pedicle screw loosening or damage to the original pedicle, the choice of augmentation materials for pedicle screw revision can have varying outcomes. In the present study, it was observed that only the pedicles repaired with CPC exhibited comparable biomechanical strength to the original pedicles. On the other hand, the pedicles repaired with ABP and DBM could not fully attain the original pedicles’ level. These findings align with the previous research conducted by Pfeifer et al. [25]. In previous experiments, our research team repeatedly pulled out pedicle screws without augmentation or revision procedures and measured the maximum pullout force. The findings revealed that the maximum re-implantation pullout force of unrepaired pedicle screws was only 33% of the original screws [13]. Moore et al. [35] conducted similar experiments and demonstrated that the maximum re-implantation pullout force of unrepaired pedicle screws was only 27.1% of the original screws. In this experiment, the maximum pullout forces of pedicle screws revised using 3 different materials were determined. The results showed that the maximum pullout forces achieved were 80.26% (ABP), 89.66% (CPC), and 64.38% (DBM) of the original pedicles, respectively. Other pedicle screw revision techniques have also been explored by researchers. For instance, using lateral entry for pedicle screw re-implantation can achieve 65% of the original screws, increasing the diameter of the revision screw can achieve 73% of the original strength, and the use of PMMA bone cement for revision can achieve 147% of the original strength [35-37]. Therefore, the technique of incorporating reinforcement materials into the pedicle for revision, particularly the use of CPC for pedicle screw repair, is worth considering. The pullout strength and failure loads of screws have been shown to be influenced by vertebral BMD [38-40]. To investigate the relationship between screw revision rate and BMD, we performed non-linear regression analysis using the methods described in the methods section. Our goal was to establish mathematical models that could describe or potentially predict the revision performance of different materials based on changes in BMD. We observed statistically significant fitting curves between revision rates and BMD for the A2 and B2 groups (Fig. 7). Based on the fitting curves, we observed that the revision rates of ABP and CPC decreased as the BMD decreased. However, an interesting finding was that for the CPC group, when the BMD was lower than 0.49 g/cm2, the decreasing trend of the revision rate slowed down or even rebounds (Fig. 7). We identified 2 intersection points on the coordinate axis, corresponding to BMD values of X1= 0.45 and X2= 0.55, respectively. Based on these findings, 2 conclusions were drawn. Firstly, CPC demonstrates higher revision efficiency in vertebrae with lower bone density. When lower bone density and the need for reinforcement material for pedicle screw revision coincide, CPC should be the preferred choice. Secondly, when BMD falls within the range of 0.45 g/cm2 to 0.55 g/cm2, ABP typically exhibits a higher revision rate than CPC. Therefore, if the vertebral BMD falls within this range and pedicle screw revision is required, ABP should be considered as the primary option. It is important to note that the sample size in this study was small, and therefore, these conclusions should be further validated by expanding the sample size and conducting follow-up experiments.
In the experiment comparing the biomechanical performance of pedicle screws augmented with 3 materials, several observations were made. During the axial pullout tests, it was found that the CPC group exhibited a significantly higher pullout force compared to both the ABP group and the DBM group. However, no significant difference in pullout force was observed between the ABP group and the DBM group. In the cyclic fatigue tests, the failure loads of the CPC group remained substantially higher than those of both the ABP group and the DBM group. When comparing the ABP group with the DBM group, it was found that the failure loads of the ABP group were higher than those of the DBM group. This result highlights that CPC exhibits the highest augmentation efficiency among the 3 materials tested, making it a favorable choice for pedicle screw augmentation. ABP, on the other hand, demonstrates superior fatigue resistance compared to DBM but does not provide as strong of a pullout force for the screw. As an alternative augmentation material, ABP can be considered. However, the use of DBM as a pedicle screw augmentation material yield limited immediate grip strength for the screw. Further research is needed to investigate whether DBM can enhance the grip strength of the screw through its osteoinductive ability during in vivo transformation.
This experiment has certain limitations that need to be acknowledged. Firstly, the sample size of vertebral bones used in this study was relatively small due to the limited availability of cadavers. While the 3 augmentation materials chosen are biocompatible and have demonstrated osteoinductive effects in vivo [26,41], it is important to note that the experiment was conducted using fresh frozen cadaveric vertebral specimens. The focus of this study was primarily on investigating the immediate stability provided by these reinforcement materials upon implantation into the vertebral pedicle. Further research is necessary to explore the in vivo transformation of these materials and whether their grip strength for screws changes after biological transformation. Providing adequate immediate grip strength following the implantation of reinforcement materials into pedicle is a crucial prerequisite for their application in pedicle screw augmentation. If immediate grip strength cannot be achieved, subsequent biological transformation processes in vivo may be hindered. Additionally, it is important to acknowledge that the cadaveric biomechanical testing employed in this study may not fully replicate the actual stress conditions experienced by pedicle screws in vivo. Therefore, further experiments, including animal studies, are required to validate the feasibility and safety of these methods before their clinical application.
CONCLUSION
Our findings indicate that ABP, CPC, and DBM can significantly improve the stability of pedicle screws in osteoporotic vertebrae when used in pedicle screw augmentation. The augmentation efficiency of these materials is ranked as follows: CPC > ABP > DBM. For revision of pedicle screws, CPC is recommended as the primary choice, with ABP as a viable alternative in select cases. However, using DBM for pedicle screw revision is not recommended based on the study’s outcomes.
Notes
Conflict of Interest
The authors have nothing to disclose.
Funding/Support
This study was supported by National Key Research and Development Plan Project of Anhui Province, China (Grant No. 2022YFC2407504) and the First Affiliated Hospital of Anhui Medical University (Grant No. 2020kj06).
Author Contribution
Conceptualization: CJ, RZ, CS; Formal Analysis: CJ, RZ, JW, BZ, HZ, LK; Investigation: CJ, JW, BZ, LZ; Methodology: CJ, RZ, LZ; Project Administration: CJ, RZ, CS; Writing – Original Draft: CJ; Writing – Review & Editing: RZ, CS.
Acknowledgements
We would like to thank for Hai Gao from the First Affiliated Hospital of USTC and Tao Xing from Children’s Hospital of Soochow University for biomechanical testing assistance.